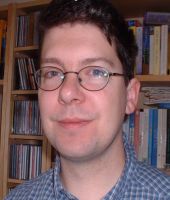 |
Dr
Daniel J Price
Department of Chemistry
University of Glasgow
Glasgow G12 8QQ
Scotland
Telephone: +44
(0) 141 330 8794
FAX: +44 (0) 330 4888
email: d.price@chem.gla.ac.uk
|
Biography
Born in Bristol Daniel Price attended St Katherine's School and Sixth Form.
In 1991 he graduated from the University of Sheffield with BSc honours in Chemistry.
He obtained a PhD (Sheffield) for research into hydrogen bond induced mesomorphism,
(a project supervised by Professor Duncan Bruce). He subsequently moved to France
to take a postdoctoral position with the late Professor Olivier Kahn at the
ICMCB in Bordeaux. This time researching a different aspect of phase behaviour;
cooperative magnetism in transition metal coordination networks. In 1995 he
returned to the UK as a postdoctoral fellow working with Professor Annie Powell
and Dr Paul Wood at the University of East Anglia. Here many new coordination
network materials were discovered using hydrothermal methods, many of which
showed complex magnetic behaviour. Daniel Price was awarded an EPSRC Advanced
Research Fellowship in 1999 to develop synthesis for hybrid inorganic-organic
materials, which can have unusual electronic and magnetic properties. The Fellowship
initially commenced at Edinburgh University before being transferred to Southampton
University in 2000. In 2004 he was appointed to a lectureship in chemistry at
the University of Glasgow.
Research Interests
Keywords: Hybrid materials, condensed matter physics, coordination
networks, magnetic interactions, spin frustration, magnetic ordering, cooperative
phenomena, phase behaviour, low dimensional systems.
Current Interests
Most generally we are interested in the complex behaviour of many body
systems having different types of interactions. In other words we are
interested in cooperativity, phase structures
and phase behaviour, or to put it another way, we are interested
in symmetry, structure, patterns,
textures, order and disorder,
phase transitions and order parameters. This is
a very far-reaching field of science, and theories of phase behaviour
are applied to such diverse things as the early history of the universe,
superconductors, fluid flow, protein folding, liquid crystals, as well
as boiling water for your cup of tea.
|
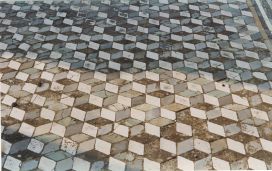
Roman mosaic - Pompeii. Three-fold symmetry can result in magnetic
spin frustration and the suppression of conventional order.
|
We enter this field as magnetochemists, using magnetic moments
from atoms with unpaired electrons as objects that can interact with each other
through a magnetic exchange interaction. We are interested in spatial and temporal
magnetic structures and the magnetic phase behaviour.
Both the detailed nature of the moments, and the coupling interaction are important,
as is the network dimension and topology. These things can be controlled to
some extent by using a "crystal engineering" approach, building specific
arrays of transition metal ions and linkers to mediate the coupling interaction.Our
aims are to form new materials which both test the current theories of solid-state
(condensed matter) physics and to form materials where very interesting and
unusual physical behaviour is expected.
An example of this is the humble antiferromagnetically coupled chain of spins.
Even such a simple system can show some bizarre behaviour. For a chain of antiferromagnetically
coupled S=1/2 ions theory says that no long-range order can exist above absolute
zero, and at low temperatures you have a compressible 1-D spin liquid like order.
By contrast if we replace the magnetic moments with an S=1 ion, the behaviour
is qualitatively different. Such a system can undergo a phase transition to
an incompressible 1-D spin liquid state, where there is quantum coherence, and
a curious combination of long-range and liquid-like order (known as hidden string
order) not seen elsewhere in nature. By contrast for the S = 2 case we know
very little about the magnetic behaviour at low temperatures. Such a magnetic
system is a clear target as it offers a rare opportunity for us experimentalists
to tell theoreticians how the world really is.
This is just one of many types of magnetic materials
which are considered as "hot topics" in the field of magnetochemistry
today.
Our research is directed at the formation of just such magnetically interesting
materials, where we control the nature of the microscopic magnetic moment
(the spins) and the structure of the extended magnetically coupled network.
We use a variety of techniques to grow colourful crystals with transition
metal network structures, where bridging ligands mediate a particular type
of interaction. Our studies include significant initial magnetic and other
characterisation of any new materials. The group makes use of a wide range
of techniques including: UV, Visible, NIR, IR, spectroscopy, TGA with EGA,
PXRD and single crystal XRD, powder and single crystal SQUID magnetometry |
|
(RSO/dc/ac- as a function of temperature, field, offset field,
drive frequency), heat capacity, neutron diffraction, MuSR, single crystal
paramagnetic NMR, NQR, Mössbauer, High field experiments, EPR, SEM,
EDAX. |
Hydrothemally grown crystals: GaNa2Ba3Fe3(ox)9
|
Recent Highlights:
Pseudo 1-D atiferromagnets
Ba4Fe4(OH)4(ox)5Cl2:
Consists of chains of hydroxide bridged iron(II) ions, that are then
connected into 3-D array by bridging oxalate ions. Magnetically there
is a strong intra-chain interaction, thus as the sample is cooled a significant
1-D correlation between spin moments develops. The system behaves with
XY anisotropy, and the experiences a crossover to conventional 3-D ordering
below 32 K.
Single crystal magnetometry, muon spin rotation, and powder neutron diffraction,
are consistent with the behaviour, and allow us to extract the thermal
evolution of the 1-D spin correlation length, as well as the sub-lattice
magnetisation order parameter.
- "Hydrothermal synthesis, X-ray structure and complex magnetic
behaviour of Ba4(C2O4)Cl2[{Fe(OH)(C2O4)}4]",
D.J. Price, S. Tripp, A.K. Powell and P.T. Wood, Chem. Euro. J.,
2001, 7, (1) 200 - 208.
|
Change in asymmetry of polarized implanted muons across the magnetic
phase transition.
|
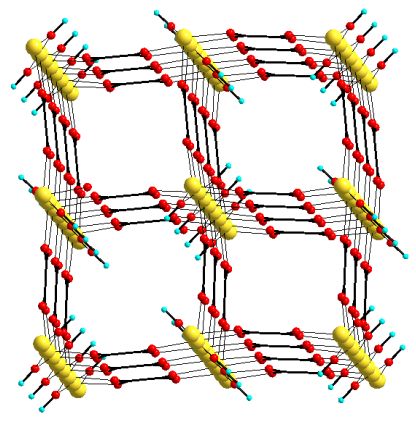
Network structure formed by Iron hydroxyl oxalate components
(Fe - Yellow).
Ni(ox)(pip): is comprised of antiferromagnetically
coupled nickel(II) oxalate chains that are magnetically well isolated
by the piperazine spacer. Ni(II) has a d8 (S = 1) electronic configuration,
and this material appears to show a Haldane phase (i.e. the unusual magnetic
order described above) at low temperatures. The crystal symmetry make
this material a particularly interesting candidate for further study as
the position of inversion centres simplifies/constrains the magnetic behaviour.
|
|
Left: structure of Ni(pip)(ox).
Below left: Thermal dependence of magnetic susceptibility.
Below right: Derived nearest neighbour pair correlation function
as a function of thermal energy.
|
|
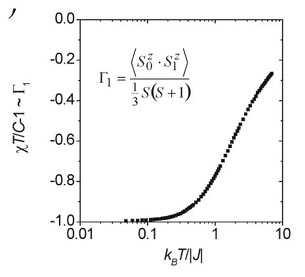 |
- "One-dimensional magnetism in new, layered structures: piperazine-linked
copper and nickel oxalate chains", T.D. Keene, H.R. Ogilvie, M.B. Hursthouse
and D.J. Price, Euro. J. Inorg. Chem., 2004, (5), 1007 - 1013.
K2M(ox)2 M=Fe, Co: The structure consists of zigzag chains of
oxalate bridged M(II) ions. The materials are isostructural and the M(II)
ion experiences a very rare trigonal prismatic coordination geometry.
The coupling in the chains is strong and antiferromagnetic, however a
weak inter-chain coupling is responsible for a magnetic phase transition
to and ordered antiferromagnet at temperatures below ~ 10 K. This is most
clearly seen in the magnetic neutron diffraction (D20, ILL Grenoble) which
develop new Bragg peaks below the critical temperature.
|
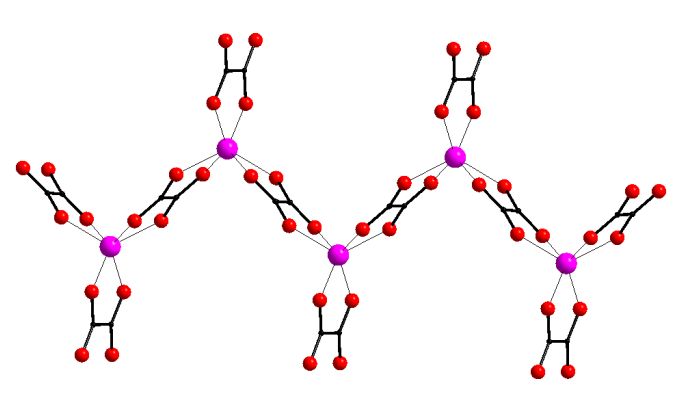
The structure of K2Co(ox)2, showing the chain of oxalate bridged Co(II)
ions with trigonal prismatic coordination.
|
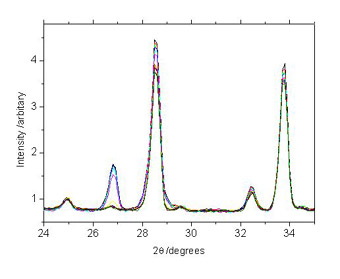
Neutron powder diffraction of K2Co(ox)2 as the sample is cooled through
a magnetic phase transition, showing the evolution of new purely magnetic
Bragg peaks.
|
- "One-dimensional magnetism in new anhydrous iron and cobalt ternary
oxaltes with rare trigonal prismatic metal coordination", M.B. Hursthouse,
M.E. Light and D.J. Price, Angew. Chem. Int. Ed., 2004, 43,
(4), 472 - 475; Also published in Angew. Chem., 2004, 116, (4),
478 - 481.
Vacancies:
Please contact Dr Price directly
for more information.
Publications
2005
- "A study of the magnetic structures of K2NiF4-type
iron(III) oxides and oxide halides, including the cobalt substituted series
Sr2Fe1-xCoxO3Cl an unusual magnetic
phase change in Sr2FeO3F", A.L. Hector, C.S. Knee,
A.I. MacDonald, D.J. Price and M.T. Weller, J. Mater. Chem., in press
2005.
- "Possible strong symmetric hydrogen bonding in disodium trihydrogen
bis(2,2'-oxydiacetate) nitrate", D.J. Price, S. Fritsch, P.T. Wood and
A.K. Powell, Acta Cryst. 2005, E61, (6), m1174 - m1177.
2004
- "Synthesis, structure and magnetism of a novel carboxylate bridged
manganese(II) sheet structure containing the first unsupported aquo bridge",
J.C. Goodwin, D.J. Price and S.L Heath, Dalton Trans. , 2004, (18),
2833 - 2835.
- "Mössbauer studies on the Fe(II) oxalate spin ladder Na2Fe2(C2O4)3(H2O)2",
J. Kreitlow, D. Baabe, A.U.B. Wolter, S. Süllow, F.J. Litterst, D.J.
Price and H.-H. Klauss, J. Mag. Mag. Mater., 2004, 272 -276,
152 - 153.
- "Ferromagnetic coupling in a heptanuclear nickel cluster with a vertex-shared
dicubane structure", T.D. Keene, M.B. Hursthouse and D.J. Price, New
J. Chem., 2004, 28, (5), 558 - 561.
- "Self-assembly of a spin-coupled octanuclearcopper(II) circular array
from a single stranded ligand", S.T. Onions, S.L. Heath, D.J. Price,
R.W. Harrington, W. Clegg and C.J. Matthews, Angew. Chem. Int. Ed.,
2004, 43, (14), 1814 - 1817; Also published in Angew. Chem.,
2004, 116, (14), 1850 - 1853.
- "[Tetra-n-butylamino(carbonato- k2O,O')-cobalt(III)
n-butylcarbamate dihydrate", T.D. Keene, M.B. Hursthouse and D.J. Price,
Acta Cryst. 2004, E60, (4), m381 - m383.
- "1,4-Diazoniabicyclo[2.2.2]octane aqua-bis(oxalato-k2O,O'-)copper(II)
dihydrate ", T.D. Keene, M.B. Hursthouse and D.J. Price, Acta Cryst.
2004, E60, (4), m378 - m380.
- "One-dimensional magnetism in new, layered structures: piperazine-linked
copper and nickel oxalate chains", T.D. Keene, H.R. Ogilvie, M.B. Hursthouse
and D.J. Price, Euro. J. Inorg. Chem., 2004, (5), 1007 - 1013.
- "Stabilisation of discreet [Cu(C2O4)2(H2O)2]2-
dianions in the solid state by an extensive hydrogen bonding network",
T.D. Keene, M.B. Hursthouse and D.J. Price, Z. Anorg. Allg. Chem.,
2004, 630, (3), 350 - 352.
- "One-dimensional magnetism in new anhydrous iron and cobalt ternary
oxaltes with rare trigonal prismatic metal coordination", M.B. Hursthouse,
M.E. Light and D.J. Price, Angew. Chem. Int. Ed., 2004, 43,
(4), 472 - 475; Also published in Angew. Chem., 2004, 116, (4),
478 - 481.
2003
- "trans-Bis(2-aminoanilinium-kN2)bis(oxalato-k2O,O'-)copper(II)",
T.D. Keene, M.B. Hursthouse and D.J. Price, Acta Cryst., 2003, E59,
(12), m1129 - m1130.
- "Two- and three-dimensional magnetic order in the layered cobalt oxychloride
Sr2CoO3Cl", C.S. Knee, D.J. Price, M.R. Lees and M.T. Weller, Phys.
Rev. B., 2003, 68, (17), 174407.
- "A self-assembled tetracopper triple-stranded helicate: Towards the
controlled synthesis of finite one-dimensional magnetic chains", C.J.
Matthews, S.T. Onions, G. Morata, L.J. Davis, S.L. Heath and D.J. Price, Angew.
Chem. Int. Ed., 2003, 42, (45), 3166 - 3169; Also published in Angew.
Chem., 2003, 115, (27), 3274 - 3277.
- "A hybrid metalloarsnate 3D framework - 1D interupted metal oxide",
R.W. Hughes, L.A. Gerrard, D.J. Price and M.T. Weller, Inorg. Chem.,
2003, 42, (13), 4160 - 4164.
- "A new series of layered transition metal oxalates: Hydrothermal synthesis,
structure and preliminary magnetic studies", D.J. Price, A.K. Powell
and P.T. Wood, Dalton Trans., 2003, (12), 2478 - 2482.
- "Synthesis, structure and magnetism of a unique dodecanuclearcopper(II)
'picture frame' held in a 4 × 4 grid-like assembly", C.J. Matthews,
S.T. Onions, G. Morata, M. Bosch Salvia, M.R.J. Elsegood and D.J. Price, Chem.
Commun., 2003, (3), 320 - 321.
- "Synthesis and structures of potassium bisdithiocarbamate salts",
D.A. Cook, S.J. Coles, M.B. Hursthouse and D.J. Price, Z. Anorg. Allg.
Chem., 2003, 629, (2), 192 - 194.
2002
- "Bipheny-3,3',5,5'-tetracarboxylic acid", S.J. Coles, R. Holmes,
M.B. Hursthouse and D.J. Price, Acta Cryst., 2002, E58, (6),
o626 - o628.
2001
- "Rational design of open framework coordination solids: Synthesis and
structure of [Co5(OH)2(1,2,4,5-(O2C)4C6H2)2(H2O)4].xH2O",
S.O.H. Gutschke, D.J. Price, A.K. Powell and P.T. Wood, Eur. J. Inorg.
Chem., 2001, (11), 2739 - 2741.
- "Hydrothermal synthesis, structure and magnetism of [Co2(OH)(1,2,3-(O2C)3C6H3)(H2O)].
H2O and [Co2(OH)(1,2,3-(O2C)3C6H3)]:
magnetic D-chains with mixed cobalt geometries", S.O.H. Gutschke, D.J.
Price, A.K. Powell and P.T. Wood, Angew. Chem. Int. Ed. Engl., 2001,
40, (10), 1920 - 1923; Also published in Angew. Chem., 2001, 113,
(10), 1974 - 1977.
- "Hydrothermal synthesis, X-ray structure and complex magnetic behaviour
of Ba4(C2O4)Cl2[{Fe(OH)(C2O4)}
4]", D.J. Price, S. Tripp, A.K. Powell and P.T. Wood, Chem.
Euro. J., 2001, 7, (1) 200 - 208.
2000
- "Na2Co2(C2O4)3(H2O)2:
Hydrothemal synthesis, structure, stability and magnetism of a new ternary
oxalate", D.J. Price, A.K. Powell and P.T. Wood, J. Chem. Soc., Dalton
Trans., 2000, (20), 3566 - 3569.
- "Trithiacyclononane as a ligand for potential technetium and rhenium
radiopharmaceuticals: Synthesis of [M(9S3)(SC2H4SC2H4S)][BF4]
(M = 99Tc, Re, 188Re) via C-S bond cleavage", G.E.D.
Mullen, P.J. Blower, D.J. Price, A.K. Powell, M.J. Howard, and M.J. Went,
Inorg. Chem., 2000, 39, (18), 4093 - 4098
- "Solvothermal construction of a coordination polymer around in-situ
generated pyroglutamic acid: preparation, crystal structure and magnetic behaviour
of [Mn(C5H6NO3)2]¥",
S.O.H. Gutschke, D.J. Price, A.K. Powell and P.T. Wood, Inorg. Chem.,
2000, 39, (16), 3705 - 3707.
- "Alkali metal tempelated assembly of an iron trigonal prism",
J. Goodwin, D.J. Price, A.K. Powell and S.L. Heath, Euro. J. Inorg.
Chem., 2000, (7), 1407 - 1410.
Pre 2000
- "Large metal clusters and lattices with analogues to biology",
D.J. Price, F. Lionti, R. Ballou, P.T. Wood and A.K. Powell, Phil. Trans.
Proc. Roy. Soc. A, 1999, 357, (1762), 3089 - 3118.
- "Hydrothermal crystallisation and X-ray structure of anhydrous strontium
oxalate", D.J. Price, A.K. Powell and P.T. Wood, Polyhedron, 1999,
18, (19), 2499 - 2503.
- "New types of metal squarates. Magnetic and multitemperature X-ray
study of di-hydroxy(m6-squarato)manganese",
D.S. Yufit, D.J. Price, S.O.H. Gutschke, P.T. Wood, A.K. Powell and J.A.K.
Howard, Chem. Commun., 1999, (16), 1561 - 1562.
- "Solvothermal synthesis of the canted antiferromagnet {K2[Co(O3PCH2N(CH2CO2)2]6.xH2O",
S.O.H. Gutschke, D.J. Price, A.K. Powell and P.T. Wood, Angew. Chem. Int.
Ed. Engl., 1999, 38, (8), 1088 - 1090; Also published in German
as "Solvothermalsynthese des verkanteten antiferromagneten {K2[Co(O3PCH2N(CH2CO2)2]6.xH2O",
S.O.H. Gutschke, D.J. Price, A.K. Powell and P.T. Wood, Angew. Chem.,
2001, 111, (8), 1158 - 1160.
- "Biomimetic control of iron oxide and hydroxide phases in the iron
oxalate system", M. Molinier, D.J. Price, P.T. Wood and A.K. Powell,
J. Chem. Soc., Dalton Trans., 1997, (21), 4061 - 4068.
- "Dc and ac magnetic properties of the two-dimensional molecular-based
ferrimagnetic materials A2M2[Cu(opba)]3.nsolv
[A+ = cation, MII = MnII or CoII,
opba = ortho-phenylenebis(oxamato), and solv = solvent molecule]",
O. Cador, D.J. Price, J. Larionova, C. Mathonière, O. Kahn and J.V.
Yakhmi, J. Mater. Chem., 1997, 7, (7), 1263 - 1270.
- "Hydrogen-bonded liquid crystals from nitrophenols and alkoxystilbazoles",
D.J. Price, K. Willis, T. Richardson, G. Ungar and D.W. Bruce, J. Mater.
Chem., 1997, 7, (6), 883 - 891.
- "Mesomorphic dithiocarbamate complexes", D.J. Price, M.A. Wali
and D.W. Bruce, Polyhedron, 1997, 16, (2), 315 - 320
- "Mesomorphism induced by hydrogen bonding between complimentary components.
Crystal and molecular structure of the adduct between 4-phenylbenzoic acid
and 4-cyano-4'-stilbazole", D.J. Price, H. Adams and D.W. Bruce, Mol.
Cryst. Liq. Cryst. A, 1996, 289, 127 - 140.
- "On the mesomorphism of hydrogen-bonded complexes formed between decyloxystilbazole
and phthalic acid", K. Willis, J.E. Luckhurst, D.J. Price, J.M.J. Fréchet,
H. Kihara, T. Kato, G. Ungar and D.W. Bruce, Liq. Cryst., 1996, 21,
(4), 585 - 587.
- "Supramolecular ferroelectric liquid crystals. Hydrogen-bonded complexes
between benzoic acids and chiral stilbazoles", H. Kihara, T. Kato, T.
Uryu, S. Ujiie, U. Kumar, J.M.J. Fréchet, D.W. Bruce and D.J. Price,
Liq. Cryst., 1996, 21, (1), 25 - 30.
- "Thermodynamics of a mixed quantum-classical Heisenberg model in two
dimensions", J. Leandri, Y. Leroyer, S.V. Meshkov, Y. Meurdesoif, O.
Kahn, B. Mombelli and D.J. Price, J. Phys.- Condens. Mat., 1996, 8,
(17), L271 - L278.
- "Hydrogen-bonded liquid crystals from alkoxystilbazoles and 3-cyanophenols:
Structural control of mesomorphism. Molecular structure of the complex between
4-cyanophenol and 4-octyloxystilbazole", K. Willis, D.J. Price, H. Adams,
G. Ungar and D.W. Bruce, J. Mater. Chem., 1995, 5, (12), 2195
- 2199.
- "An investigation of the hydrogen bonding in liquid crystal systems
using variable-temperature electronic spectroscopy", D.J. Price, T. Richardson
and D.W. Bruce, J. Chem. Soc., Chem. Commun., 1995, (18), 1911 - 1912.
- "Mesophase order of a new smectic paramagnetic copper complex detected
by EPR", J.I. Martinez, D.W. Bruce, D.J. Price and P.J. Alonso, Liq.
Cryst., 1995, 19, (1), 127 - 132.
- "Liquid crystalline hydrogen-bonded complexes formed between alkoxystilbazoles
and phenols", D.W. Bruce and D.J. Price, Adv. Mater. Opt. Electron.,
1994, 4, 273.
Return to Chemistry Staff Page.